Working memory is a fundamental cognitive process that allows us to hold on to information temporarily as it comes through our senses. Like a small holding bin, working memory enables us to transiently store information, such as the name of a person we’ve just met, a grocery list or facts that we’ve just learnt at a lecture. As with a small holding bin, however, it has a limited capacity and can store only a few memories at a time. Working memory is also remarkably fragile. Even minor distractors can often lead memories to seemingly disappear without a trace: think of how easily we forget the name of a person we’ve just met, after changing the topic of conversation. Although this core cognitive process has long been the topic of intense interest and investigation by neuroscientists, how the human brain manages information about items stored in working memory has remained poorly understood. Writing in Nature, Daume et al.1 report their analyses of single neurons and neural populations in people participating in a working-memory task, and provide fresh insights into how the human brain represents and controls the maintenance of information in working memory.
Read the paper: Control of working memory by phase–amplitude coupling of human hippocampal neurons
Two key areas of the brain — the medial temporal lobe and the frontal lobe — have emerged as candidate players in working memory. In humans, for example, memory-specific activity patterns have been observed in neurons in the medial temporal lobe, which suggests that they have a role in memory storage2–4. Other neurons, in parts of the frontal lobe, have been implicated in cognitive processes such as attention and maintenance of working memory5–7. In the current study, Daume and colleagues investigated how these areas interact to control working memory. They did this by recording the activities of individual neurons using specialized intracranial electrodes, which were inserted in the frontal and temporal lobes of volunteers as part of planned neurosurgical care for monitoring epilepsy. This approach allowed the team to track single-neuron activities as the participants held several items (in this case, pictures) in working memory.
The authors found that certain neurons synchronized their firing activity in a process called phase–amplitude coupling, whereby the phase of a slower electrophysiological oscillation couples with the amplitude of a faster oscillation. Specifically, they identified subpopulations of neurons in the temporal lobe whose activity reflected the coupling between the phase of theta waves (oscillating at a frequency of 3 to 7 hertz) and the amplitude of gamma waves (oscillating at 30 to 140 Hz). Moreover, the degree to which these neurons coordinated their activity with the theta activity of neurons in the frontal lobe depended on the number of items that had to be held in working memory, and therefore reflected the degree of cognitive control that needed to be exerted (Fig. 1).
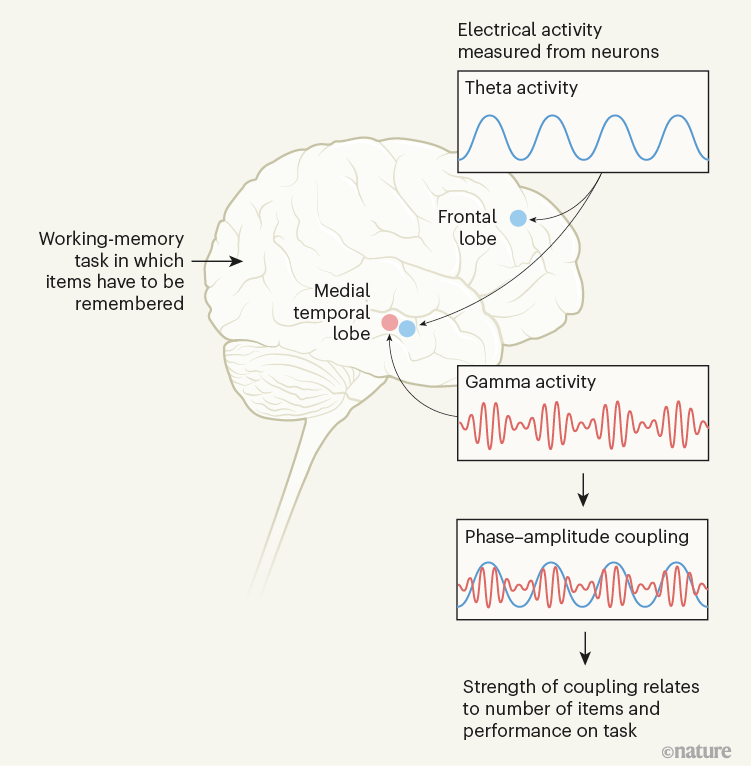
Figure 1 | Working memory in the human brain. Daume et al.1 recorded neuronal activity in the brains of volunteers as they performed a task in which they had to hold one or more pictures (referred to as items) in working memory. The authors identified neurons in the medial temporal lobe whose phase of slower (theta, 3–7 hertz) activity was coupled to the amplitude of faster (gamma, 30–140 Hz) activity. Interestingly, they found that these neurons coordinated their activities with the theta activity of neurons in the frontal lobe, and that the strength of this coordinated activity depended on the number of items that a participant had to remember, and how accurately they remembered them. The findings suggest that phase–amplitude coupling enables the frontal areas of the brain to exert control over temporal areas in which information about items held in working memory is processed and maintained.
Notably, the authors also found that neurons that took part in phase-amplitude coupling were mostly distinct from ‘category’ neurons, which encoded information about the specific items being held in working memory. This provides evidence of a division between neurons that store information temporarily and those that control its maintenance. Finally, Daume et al. showed that neurons involved in phase–amplitude coupling influenced the activity patterns of the neuronal population. These patterns correlated with how accurately the information about the items was held in memory and therefore how well the participants performed in the task.
How speech is produced and perceived in the human cortex
Together, these findings are striking, because they suggest that working memory is supported by a multicomponent mechanism whereby frontal areas exert control over the maintenance of working memory in areas where memories are stored, such as the medial temporal lobe. The results also prompt many further lines of investigation. Why do humans have a limited working-memory capacity, and why do certain individuals have a better working memory than others do? How might different forms of information, such as names, faces and pictures, be optimally stored? Insights into how the frontal lobe exerts control over working memory could also indicate how memory failure originates, and could make way for new avenues of research aimed at developing treatments for conditions such as Alzheimer’s disease, in which working memory is often affected8,9.
This understanding of working memory could open up many exciting prospects in the field of human neurophysiology. These might include the development of closed-loop neural prosthetics (self-modulating devices designed to replace a lost neurological function) that could modulate or enhance working memory. For example, it would be interesting to explore whether theta–gamma phase–amplitude coupling can be enhanced using precisely aligned deep-brain stimulations, or whether working memory can be improved in individuals with neurodegenerative disorders or conditions associated with traumatic brain injury.
Future research could also examine whether it is possible to limit the effects of distractors, or enhance working-memory capacity, through biofeedback — a therapeutic technique in which sensors are used to enable a person to monitor physiological changes, including patterns of neurological activity, in their own body. Daume and colleagues’ study provides fertile ground on which to begin investigating these possibilities — including how to remember the name of that person you just met.