Abstract
Biological systems can create materials with intricate structures and specialized functions. In comparison, precise control of structures in human-made materials has been challenging. Here we report on insect cuticle peptides that spontaneously form nanocapsules through a single-step solvent exchange process, where the concentration gradient resulting from the mixing of water and acetone drives the localization and self-assembly of the peptides into hollow nanocapsules. The underlying driving force is found to be the intrinsic affinity of the peptides for a particular solvent concentration, while the diffusion of water and acetone creates a gradient interface that triggers peptide localization and self-assembly. This gradient-mediated self-assembly offers a transformative pathway towards simple generation of drug delivery systems based on peptide nanocapsules.
This is a preview of subscription content, access via your institution
Access options
Access Nature and 54 other Nature Portfolio journals
Get Nature+, our best-value online-access subscription
$29.99 / 30 days
cancel any time
Subscribe to this journal
Receive 12 print issues and online access
$259.00 per year
only $21.58 per issue
Buy this article
- Purchase on Springer Link
- Instant access to full article PDF
Prices may be subject to local taxes which are calculated during checkout
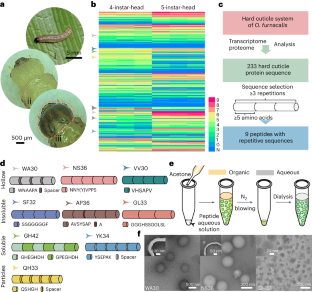





Similar content being viewed by others
Data availability
The data that support the plots within this paper and other findings of this study are available via DR-NTU at https://doi.org/10.21979/N9/DJV2BM (ref. 58). The data that support the findings of this study are also available from the corresponding authors upon reasonable request. Source data are provided with this paper.
Code availability
All code and scripts used in this study are available from the corresponding authors upon reasonable request.
References
Bar-Cohen, Y. Biomimetics—using nature to inspire human innovation. Bioinspir. Biomim. 1, P1 (2006).
Liu, Z., Meyers, M. A., Zhang, Z. & Ritchie, R. O. Functional gradients and heterogeneities in biological materials: design principles, functions, and bioinspired applications. Prog. Mater. Sci. 88, 467–498 (2017).
Waite, J. H., Lichtenegger, H. C., Stucky, G. D. & Hansma, P. Exploring molecular and mechanical gradients in structural bioscaffolds. Biochemistry 43, 7653–7662 (2004).
Miserez, A., Li, Y., Waite, J. H. & Zok, F. Jumbo squid beaks: inspiration for design of robust organic composites. Acta Biomater. 3, 139–149 (2007).
Ho, S. P., Marshall, S. J., Ryder, M. I. & Marshall, G. W. The tooth attachment mechanism defined by structure, chemical composition and mechanical properties of collagen fibers in the periodontium. Biomaterials 28, 5238–5245 (2007).
Meyers, M. A., McKittrick, J. & Chen, P.-Y. Structural biological materials: critical mechanics–materials connections. Science 339, 773–779 (2013).
Fratzl, P. & Weinkamer, R. Nature’s hierarchical materials. Prog. Mater. Sci. 52, 1263–1334 (2007).
Heinemann, F., Launspach, M., Gries, K. & Fritz, M. Gastropod nacre: structure, properties and growth—biological, chemical and physical basics. Biophys. Chem. 153, 126–153 (2011).
Chen, P.-Y., Stokes, A. & McKittrick, J. Comparison of the structure and mechanical properties of bovine femur bone and antler of the North American elk (Cervus elaphus canadensis). Acta Biomater. 5, 693–706 (2009).
Silva, E. C. N., Walters, M. C. & Paulino, G. H. Modeling bamboo as a functionally graded material: lessons for the analysis of affordable materials. J. Mater. Sci. 41, 6991–7004 (2006).
Korevaar, P. A., Schaefer, C., de Greef, T. F. & Meijer, E. Controlling chemical self-assembly by solvent-dependent dynamics. J. Am. Chem. Soc. 134, 13482–13491 (2012).
Wang, J. et al. Trace solvent as a predominant factor to tune dipeptide self-assembly. ACS Nano 10, 2138–2143 (2016).
Hartgerink, J. D., Beniash, E. & Stupp, S. I. Self-assembly and mineralization of peptide-amphiphile nanofibers. Science 294, 1684–1688 (2001).
Lee, B. K., Yun, Y. & Park, K. PLA micro- and nano-particles. Adv. Drug Deliv. Rev. 107, 176–191 (2016).
El-Sheikh, S., El-Sherbiny, S., Barhoum, A. & Deng, Y. Effects of cationic surfactant during the precipitation of calcium carbonate nano-particles on their size, morphology, and other characteristics. Colloids Surf. A 422, 44–49 (2013).
Cheng, J. et al. Formulation of functionalized PLGA–PEG nanoparticles for in vivo targeted drug delivery. Biomaterials 28, 869–876 (2007).
Moreno‐Alcántar, G. et al. Solvent‐driven supramolecular wrapping of self‐assembled structures. Angew. Chem. Int. Ed. Engl. 133, 5467–5473 (2021).
Te Brinke, E. et al. Dissipative adaptation in driven self-assembly leading to self-dividing fibrils. Nat. Nanotechnol. 13, 849–855 (2018).
Albert, J. N. & Epps, T. H. III Self-assembly of block copolymer thin films. Mater. Today 13, 24–33 (2010).
Knowles, T. P., Oppenheim, T. W., Buell, A. K., Chirgadze, D. Y. & Welland, M. E. Nanostructured films from hierarchical self-assembly of amyloidogenic proteins. Nat. Nanotechnol. 5, 204–207 (2010).
Rideau, E., Wurm, F. R. & Landfester, K. Self‐assembly of giant unilamellar vesicles by film hydration methodologies. Adv. Biosyst. 3, 1800324 (2019).
Blanazs, A., Armes, S. P. & Ryan, A. J. Self‐assembled block copolymer aggregates: from micelles to vesicles and their biological applications. Macromol. Rapid Commun. 30, 267–277 (2009).
Cui, H., Chen, Z., Zhong, S., Wooley, K. L. & Pochan, D. J. Block copolymer assembly via kinetic control. Science 317, 647–650 (2007).
Rabani, E., Reichman, D. R., Geissler, P. L. & Brus, L. E. Drying-mediated self-assembly of nanoparticles. Nature 426, 271–274 (2003).
Sorrenti, A., Leira-Iglesias, J., Markvoort, A. J., de Greef, T. F. & Hermans, T. M. Non-equilibrium supramolecular polymerization. Chem. Soc. Rev. 46, 5476–5490 (2017).
Rivas, C. J. M. et al. Nanoprecipitation process: from encapsulation to drug delivery. Int. J. Pharm. 532, 66–81 (2017).
Andersen, S. O. Biochemistry of insect cuticle. Annu. Rev. Entomol. 24, 29–59 (1979).
Gopalan Nair, K. & Dufresne, A. Crab shell chitin whisker reinforced natural rubber nanocomposites. 1. Processing and swelling behavior. Biomacromolecules 4, 657–665 (2003).
Bogatyreva, N. S., Finkelstein, A. V. & Galzitskaya, O. V. Trend of amino acid composition of proteins of different taxa. J. Bioinform. Comput. Biol. 4, 597–608 (2006).
Willis, J. H. Structural cuticular proteins from arthropods: annotation, nomenclature, and sequence characteristics in the genomics era. Insect Biochem. Mol. Biol. 40, 189–204 (2010).
Kajava, A. V. Tandem repeats in proteins: from sequence to structure. J. Struct. Biol. 179, 279–288 (2012).
Lebouille, J., Stepanyan, R., Slot, J., Stuart, M. C. & Tuinier, R. Nanoprecipitation of polymers in a bad solvent. Colloids Surf. A 460, 225–235 (2014).
Aryal, S., Hu, C.-M. J. & Zhang, L. Polymer–cisplatin conjugate nanoparticles for acid-responsive drug delivery. ACS Nano 4, 251–258 (2010).
Hyde, C., Johnson, T. & Sheppard, R. Internal aggregation during solid phase peptide synthesis. Dimethyl sulfoxide as a powerful dissociating solvent. J. Chem. Soc. Chem. Commun. https://doi.org/10.1039/C39920001573 (1992).
Srivastava, K. R., Kumar, A., Goyal, B. & Durani, S. Stereochemistry and solvent role in protein folding: nuclear magnetic resonance and molecular dynamics studies of poly-l and alternating-l, d homopolypeptides in dimethyl sulfoxide. J. Phys. Chem. B 115, 6700–6708 (2011).
Mendoza-Novelo, B., Mata-Mata, J. L., Vega-González, A., Cauich-Rodríguez, J. V. & Marcos-Fernández, Á. Synthesis and characterization of protected oligourethanes as crosslinkers of collagen-based scaffolds. J. Mater. Chem. B 2, 2874–2882 (2014).
Gu, L., Jiang, Y. & Hu, J. Scalable spider‐silk‐like supertough fibers using a pseudoprotein polymer. Adv. Mater. 31, 1904311 (2019).
Annabi, N. et al. Synthesis of highly porous crosslinked elastin hydrogels and their interaction with fibroblasts in vitro. Biomaterials 30, 4550–4557 (2009).
Nowatzki, P. J. & Tirrell, D. A. Physical properties of artificial extracellular matrix protein films prepared by isocyanate crosslinking. Biomaterials 25, 1261–1267 (2004).
Ramos, R. et al. Nanocapsules produced by nanoprecipitation of designed suckerin-silk fusion proteins. ACS Macro Lett. 10, 628–634 (2021).
Zhang, L. et al. Microfluidic synthesis of rigid nanovesicles for hydrophilic reagents delivery. Angew. Chem. Int. Ed. Engl. 127, 4024–4028 (2015).
Wang, Z., Rutjes, F. P. & van Hest, J. C. pH responsive polymersome Pickering emulsion for simple and efficient Janus polymersome fabrication. Chem. Commun. 50, 14550–14553 (2014).
Reuvers, A. & Smolders, C. Formation of membranes by means of immersion precipitation: part II. The mechanism of formation of membranes prepared from the system cellulose acetate-acetone-water. J. Membr. Sci. 34, 67–86 (1987).
Taylor, N. O., Wei, M.-T., Stone, H. A. & Brangwynne, C. P. Quantifying dynamics in phase-separated condensates using fluorescence recovery after photobleaching. Biophys. J. 117, 1285–1300 (2019).
Ray, S. et al. Mass photometric detection and quantification of nanoscale α-synuclein phase separation. Nat. Chem. 15, 1306–1316 (2023).
Greenfield, N. J. Analysis of the kinetics of folding of proteins and peptides using circular dichroism. Nat. Protoc. 1, 2891–2899 (2006).
Vögeli, B., Kazemi, S., Güntert, P. & Riek, R. Spatial elucidation of motion in proteins by ensemble-based structure calculation using exact NOEs. Nat. Struct. Mol. Biol. 19, 1053–1057 (2012).
Ulijn, R. V. & Smith, A. M. Designing peptide based nanomaterials. Chem. Soc. Rev. 37, 664–675 (2008).
Nauman, J. V., Campbell, P. G., Lanni, F. & Anderson, J. L. Diffusion of insulin-like growth factor-I and ribonuclease through fibrin gels. Biophys. J. 92, 4444–4450 (2007).
Cui, H., Webber, M. J. & Stupp, S. I. Self‐assembly of peptide amphiphiles: from molecules to nanostructures to biomaterials. Biopolymers 94, 1–18 (2010).
Tian, Y., Tirrell, M. V. & LaBelle, J. L. Harnessing the therapeutic potential of biomacromolecules through intracellular delivery of nucleic acids, peptides, and proteins. Adv. Healthc. Mater. 11, 2270066 (2022).
Tang, L. et al. Investigating the optimal size of anticancer nanomedicine. Proc. Natl Acad. Sci. USA 111, 15344–15349 (2014).
Midoux, P., Pichon, C., Yaouanc, J. J. & Jaffrès, P. A. Chemical vectors for gene delivery: a current review on polymers, peptides and lipids containing histidine or imidazole as nucleic acids carriers. Br. J. Pharmacol. 157, 166–178 (2009).
Xiang, Z. et al. Adding an unnatural covalent bond to proteins through proximity-enhanced bioreactivity. Nat. Methods 10, 885–888 (2013).
Ruoslahti, E. & Pierschbacher, M. D. New perspectives in cell adhesion: RGD and integrins. Science 238, 491–497 (1987).
Naldini, L. Gene therapy returns to centre stage. Nature 526, 351–360 (2015).
Pardi, N. et al. Administration of nucleoside-modified mRNA encoding broadly neutralizing antibody protects humanized mice from HIV-1 challenge. Nat. Commun. 8, 14630 (2017).
Haopeng, L. et al. Data for: harnessing gradients for self-assembly of peptide-based nanocapsules: a pathway to advanced drug delivery systems. DR-NTU (Data) https://doi.org/10.21979/N9/DJV2BM (2023).
Acknowledgements
H.L., X.H. and J.Y acknowledge support from the Singapore National Research Fellowship (NRF-NRFF11-2019-0004) and the Singapore Ministry of Education (MOE) Tier 2 Grant (MOE-T2EP30220-0006). T.L. acknowledges support from the National Natural Science Foundation of China (31871959) and the National Key R&D Program of China (2022YFD1700200). Q.Y. acknowledges support from the National Natural Science Foundation of China (32161133010), the National Key R&D Program of China (2022YFD1700200) and the Shenzhen Science and Technology Program (KQTD20180411143628272). X.Q. and H.G. acknowledge support from the Singapore Ministry of Education (MOE) under its Academic Research Fund Tier 1 award no. RG138/20, no. RG135/22 and a start-up grant from Nanyang Technological University, Singapore and A*STAR, Singapore. G.Z and H.G. acknowledge funding support from the Ministry of Education in Singapore under grant MOE-MOET32022-0002. A.M. acknowledges support from the Singapore Ministry of Education (MOE) through an Academic Research (AcRF) Tier 3 grant (Grant No. MOE 2019-T3-1-012). We acknowledge the Facility for Analysis, Characterisation, Testing and Simulation (FACTS) and NTU Institute of Structural Biology (NISB), Nanyang Technological University, Singapore, for use of their HR-TEM, Cryo-TEM and NMR facilities. Molecular dynamics simulations reported were performed on resources provided by the High Performance Computing Centre at Nanyang Technological University, Singapore, and the National Supercomputing Centre, Singapore (http://www.nscc.sg).
Author information
Authors and Affiliations
Contributions
T.L., Q.Y., H.G. and J.Y. designed the study. Q.Y. conceptualized and supported the study. H.L., H.M., X.H., H.Q., F.Y., T.L. and J.Y. conducted the experiments. H.L. performed the experiment and analysis. X.Q. performed the modelling, simulations and analysis. H.L. and X.Q. collected the data. H.L., X.Q., H.M., X.H., H.Q., G.Z., A.M., Q.Y., T.L., H.G. and J.Y. analysed and interpreted the data. The paper was written through contributions of all authors. All authors have given approval of the final version of the paper for submission.
Corresponding authors
Ethics declarations
Competing interests
J.Y. and H.L. have filed a Singapore provisional patent application number 10202301815S.
Peer review
Peer review information
Nature Nanotechnology thanks Debasish Haldar and the other, anonymous, reviewer(s) for their contribution to the peer review of this work.
Additional information
Publisher’s note Springer Nature remains neutral with regard to jurisdictional claims in published maps and institutional affiliations.
Supplementary information
Supplementary Information
Supplementary Methods, Tables 1–7 and Figs. 1–45.
Source data
Source Data Fig. 1
Statistical source data.
Source Data Fig. 2
Statistical source data.
Source Data Fig. 3
Statistical source data.
Source Data Fig. 4
Statistical source data.
Source Data Fig. 5
Statistical source data.
Source Data Fig. 6
Statistical source data.
Rights and permissions
Springer Nature or its licensor (e.g. a society or other partner) holds exclusive rights to this article under a publishing agreement with the author(s) or other rightsholder(s); author self-archiving of the accepted manuscript version of this article is solely governed by the terms of such publishing agreement and applicable law.
About this article
Cite this article
Li, H., Qian, X., Mohanram, H. et al. Self-assembly of peptide nanocapsules by a solvent concentration gradient. Nat. Nanotechnol. (2024). https://doi.org/10.1038/s41565-024-01654-w
Received:
Accepted:
Published:
DOI: https://doi.org/10.1038/s41565-024-01654-w